Strength Testing in Cycling: Prevention and performance
Available in:
EN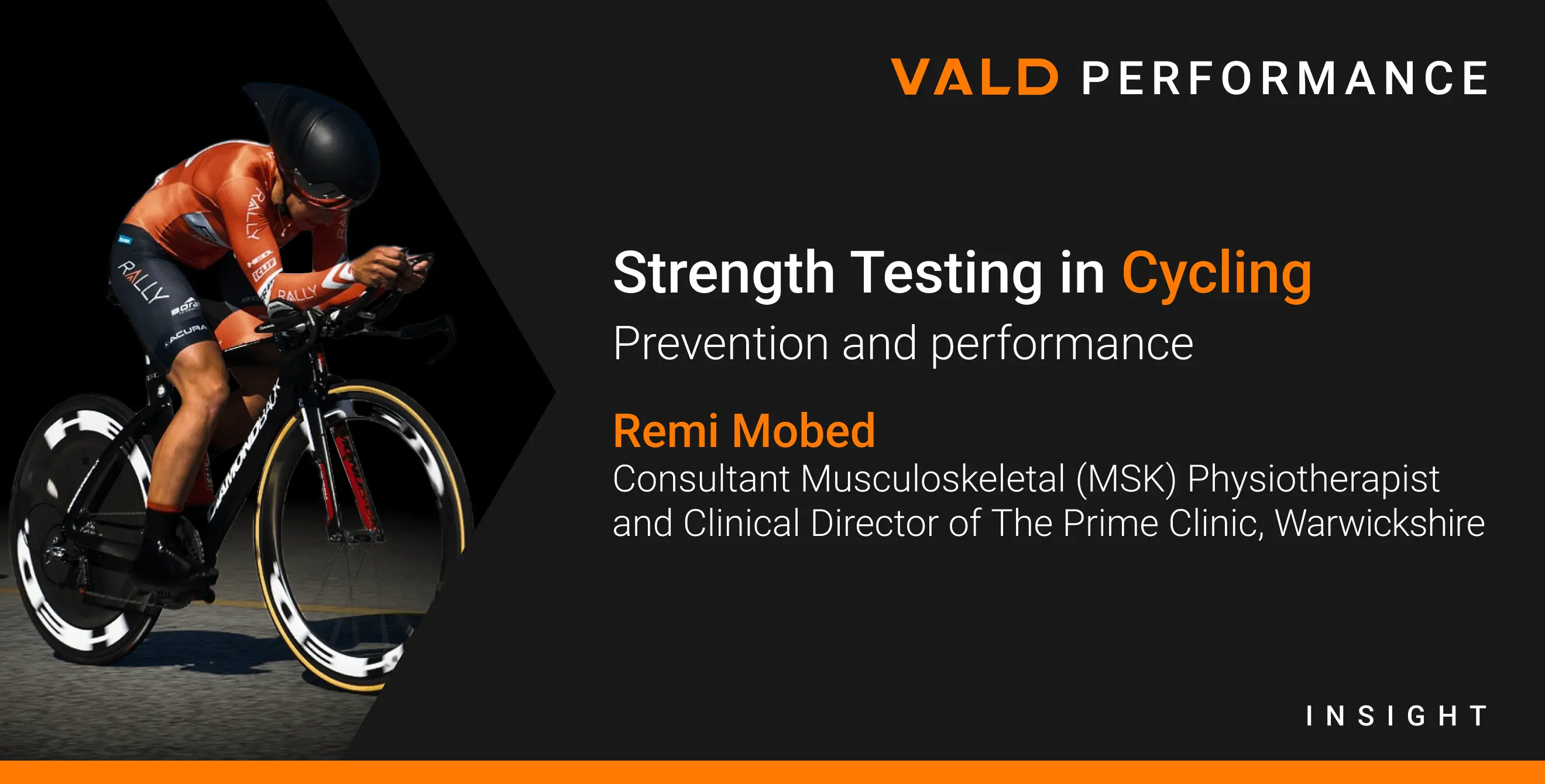
About the Author
Remi Mobed is a Consultant Musculoskeletal (MSK) Physiotherapist who is currently the rehabilitation lead for the INEOS Grenadiers pro-cycling team and Clinical Director of The Prime Clinic, Warwickshire.
With a deep understanding of cycling performance and rehabilitation, Mobed highlights how cycling – whether for competition, leisure or fitness – demands a blend of muscular strength, power and endurance. To meet these demands, muscle performance testing plays a pivotal role in identifying a cyclist's strengths and areas for improvement, formulating tailored training programs and preventing injuries.
This article delves into the significance of muscle strength and power testing in cycling, using VALD systems to assess the primary muscles involved. It also explores common testing methods and how cyclists can utilize these insights to boost performance and minimize injury risks.
The Significance of Muscle Strength in Cycling
Cycling efficiency and performance are not solely contingent on cardiovascular fitness. Muscular strength, particularly in the lower body, is paramount for power generation, speed maintenance and overall bike control.
Muscular strength…is paramount for power generation, speed maintenance and overall bike control.
In cycling, stronger muscles often lead to enhanced endurance by lowering the relative force demands required to maintain a given output, allowing cyclists to maintain optimal performance levels for extended periods.
Furthermore, muscular strengthening is critical in the prevention of overuse injuries, as variability in training loads helps to reduce the monotonous and repetitive strain cycling imposes on the body.
Key Muscle Groups in Cycling: A scientific overview
Cycling relies on the coordinated activation of multiple muscle groups to generate and sustain power while maintaining stability. Therefore, identifying the primary muscles involved is essential for optimizing training and performance assessments. The key muscle groups include:
Quadriceps Femoris: The quadriceps are the primary drivers of the pedal downstroke (Diefenthaeler et al., 2011). They play a critical role in knee extension, which is pivotal during the power phase of the pedaling cycle. Their activity is highest during the initial 90° of the crank rotation (da Silva et al., 2016).
Hamstrings: The hamstrings are active near the end of extension and during the extension-to-flexion transition. As the primary knee flexors, they help drive the upward stroke and maintain smooth pedaling mechanics.
Gluteal Muscles: The gluteus maximus contributes significantly to hip extension, propelling the cyclist forward. These hip extensors produce the greatest forces during the beginning of the downstroke in cycling.
Gastrocnemius and Soleus (Calf Muscles): The calf muscles contribute around 20% of pedaling power. They activate shortly after the tibialis anterior ceases to function at approximately 30° and continue working until 270°, pressing the foot downward on the pedal until knee flexion begins (Holliday et al., 2023).
Hip Flexors: The hip flexors work to drive the pedal rearward near the bottom dead center and to lift the pedal during a large portion of the recovery phase (So et al., 2005).
Core Musculature: Core muscles like the rectus abdominis, obliques, and erector spinae stabilize posture, reduce energy loss and improve balance, especially during intense or off-road cycling.
Technology enables practitioners to develop a better understanding of the biomechanics and functional roles of these muscle groups, ultimately improving the strength training programs of athletes that have commonly been underserved in the weight room.
Common Methods for Muscle Strength Testing
1. Individual Muscle Tests: Strength of specific muscles and muscle groups related to cycling action and previous injury. Commonly assessed groups are the quadriceps, hamstrings, gluteals and calves.
2. Compound Isometric Testing: Assessment of lower body absolute strength. Tests such as isometric mid-thigh pull (IMTP) and isometric squat (Iso Squat) are used because isometric tests are safer than one-repetition maximum (1RM) testing and less fatiguing for the athlete (Blazevich et al., 2002).
Stone et al. (2004) highlight the crucial role of isometric maximum strength (IMTP) in sprint cycling performance. Their findings suggest that greater maximum strength and the ability to produce force rapidly are strongly associated with enhanced pedaling power and speed, especially during explosive efforts like sprints.
…greater maximum strength and the ability to produce force rapidly are strongly associated with enhanced pedaling power and speed…
3. Force Plate Jump Testing: While not cycling-specific, the vertical jump test is an indirect measure of lower body explosive power, beneficial for cyclists needing quick bursts of speed. Common tests are the countermovement jump (CMJ) and squat jump (SJ).
Vandewalle et al. (1987) examined the force-velocity relationship on a cycle ergometer and its correlation with vertical jump height among athletes from various sports. Their findings indicated a significant relationship between vertical jump height and maximal anaerobic power (Wmax) relative to body mass, suggesting that athletes with higher vertical jump heights exhibited greater cycling power outputs.
…athletes with higher vertical jump heights tend to exhibit greater cycling power outputs.
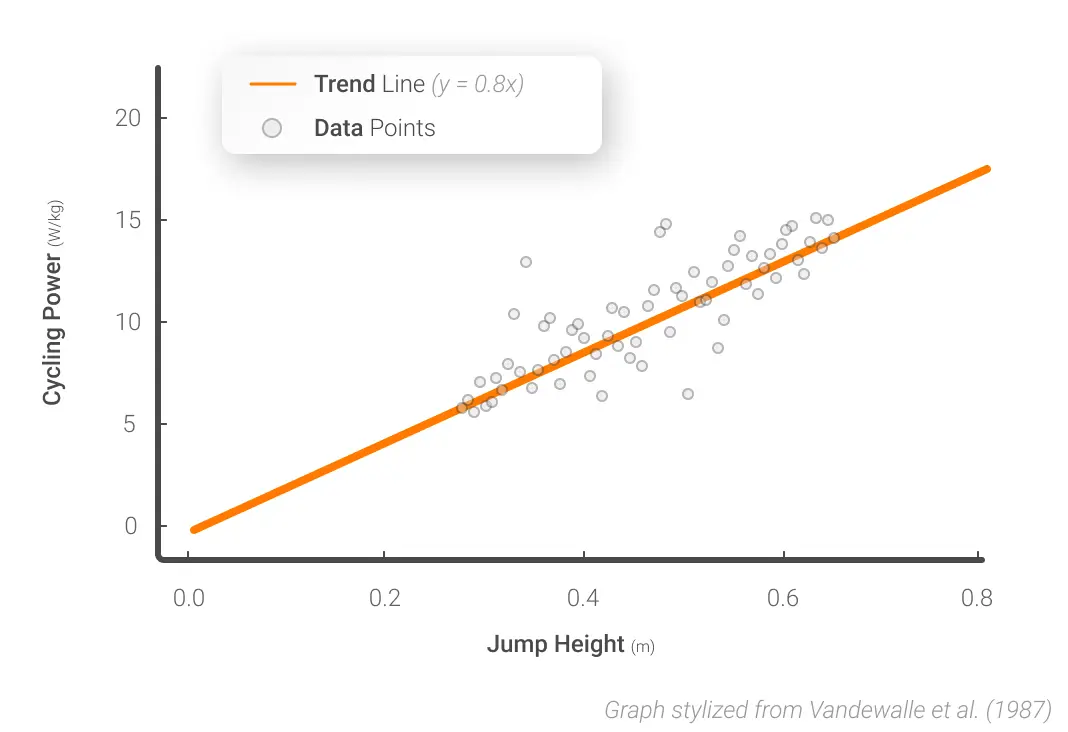
Simulated correlation between jump power from a CMJ and cycling power from a Wingate test.
4. Wingate Test: Although primarily assessing anaerobic capacity, the Wingate test, a maximal effort cycling sprint against bodyweight-normalized resistance, can also provide insights into muscular endurance and power, especially in sprint cyclists.
Translating Test Results into Performance Gains
The data gathered from muscle strength testing should inform a cyclist's training regimen. For instance, if testing reveals significant strength imbalances between the quadriceps and hamstrings, targeted resistance training can help correct this imbalance, leading to better pedal efficiency and reduced injury risk.
Moreover, identifying specific muscle weaknesses enables cyclists to focus their strength training more effectively, ensuring all relevant muscle groups receive adequate attention.
With the increasing use of power meters and cycle ergometers, practitioners are now examining the relationship between on-bike and off-bike measures of power and force expression.
Advancements in technology that enable the assessment of force distribution (e.g., left vs. right side) during cycling have opened the door to exploring similarities between cycling-specific measures and those observed in other tasks.
Injury Prevention Through Strength Testing
One of the most valuable benefits of muscle strength testing in cycling is injury prevention. By pinpointing muscle weaknesses and imbalances early, cyclists can address these issues through targeted exercises, reducing the risk of common cycling injuries such as knee pain, lower back pain and muscle strains.
…muscle strength testing…[helps pinpoint] weaknesses and imbalances early…reducing the risk of common cycling injuries such as knee pain, lower back issues and muscle strains.
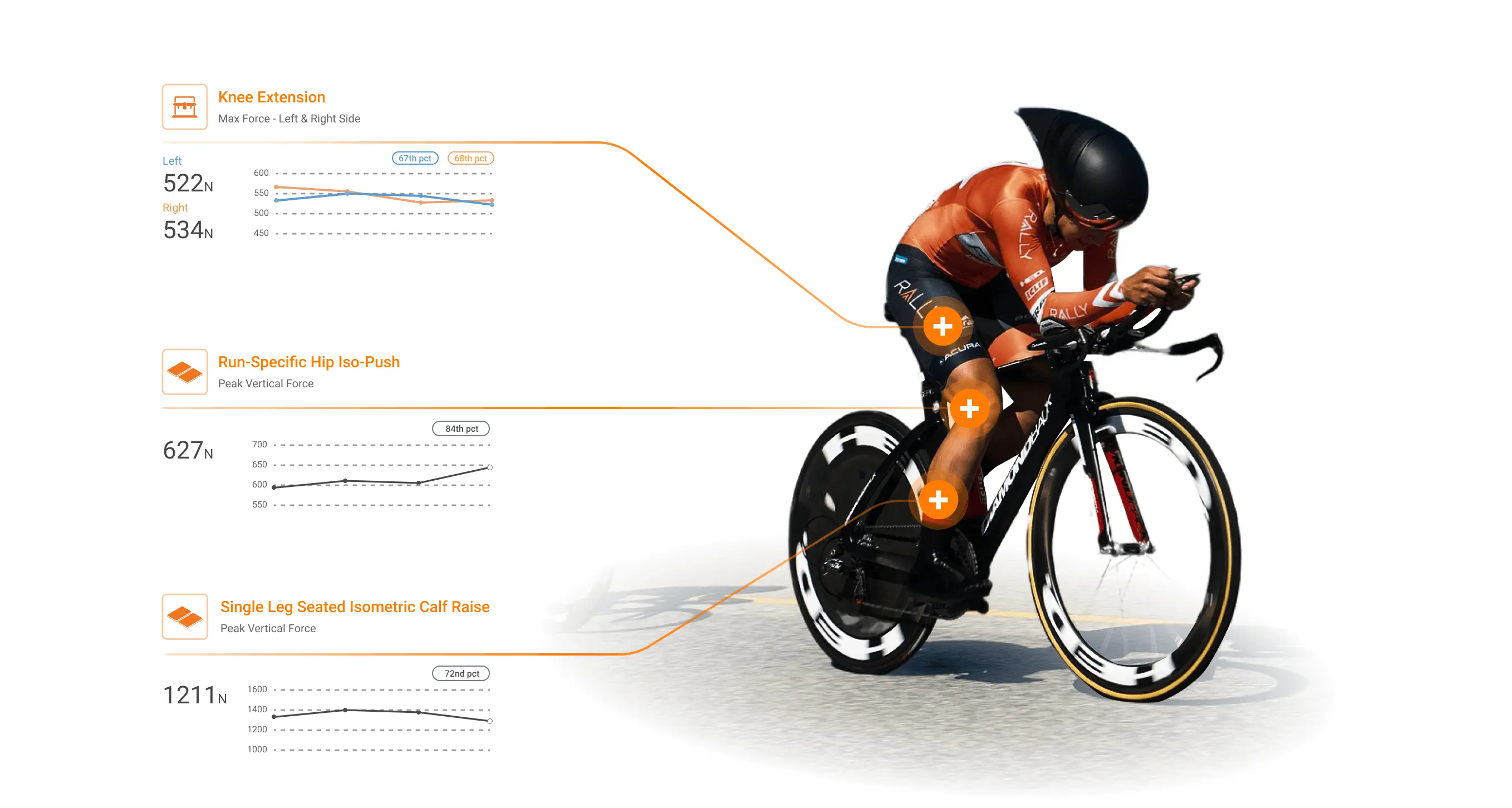
Cycling athlete racing with technology-enabled performance measures of key tests displayed.
Regular strength testing, therefore, not only contributes to enhancing performance but also plays a crucial role in maintaining a cyclist's overall health and longevity in the sport.
Baseline Testing
Gathering baselines is essential when testing athletes to enable practitioners to understand where their strengths and limitations lie.
Typically, a cyclist will go through a full battery of initial tests to establish their baseline measures and create a starting point for their training program. Having this data is pivotal to set targets for the future and to draw a line in the sand if injuries occur as practitioners are able to identify when they are back (or close) to the previous state.
Gathering baselines is essential…to set targets for the future and to draw a line in the sand if injuries occur…
To establish these baselines, practitioners use objective strength and power assessments, such as those included in VALD's testing options.
VALD Testing Options | ||
---|---|---|
Muscular Quality | VALD System | Test Types |
Strength | ForceDecks | IMTP, Iso Squat, Hip Iso-Push, Ankle Iso-Push |
ForceFrame | Hip Adduction & Abduction | |
NordBord | Nordic, ISO Prone | |
DynaMo | Knee Flexion & Extension, Core | |
Power | ForceDecks | CMJ, Squat Jump, Drop Jump |
Normative Values in Performance Testing
Normative values play a crucial role in interpreting muscle strength test results, helping practitioners benchmark individual performance against population standards. Below are some examples of normative data for muscle strength and power tests in males and females.
Vertical Jump Test (CMJ)
Cyclists, whether novice or competitive, tend to score lower on vertical jump tests, averaging around 29 ± 6cm, compared to strength-trained individuals who achieve approximately 48.2 ± 2.8cm and even untrained individuals who average 33.7 ± 2.3cm (Jongerius, 2018).
This aligns with other studies on endurance athletes, such as long-distance runners, who also perform worse on jump tests than untrained populations (27.8 ± 4.3cm vs. 37.3 ± 3.1cm; Kubo et al., 2000).
IMTP
Males: Peak force ~3.5-4.0 times bodyweight for elite sprint cyclists; ~2.5-3.0 times bodyweight for trained individuals.
Females: Peak force ~2.5-3.0 times bodyweight for elite sprint cyclists; ~1.8-2.5 times bodyweight for trained individuals.
Wingate Test (Peak Power Output)
Males: 12-15 Watts/kg bodyweight for elite cyclists; 8-10 Watts/kg for trained individuals.
Females: 9-12 Watts/kg bodyweight for elite cyclists; 6-8 Watts/kg for trained individuals.
These values provide a reference point for evaluating performance. For example, a male cyclist achieving a peak force of 2.0 times bodyweight in the IMTP may need to improve lower-body strength to meet the demands of elite competition.
Similarly, normative datasets provided in VALD Hub can help practitioners discern quality benchmarks for isolated strength measures such as the Nordic hamstring curl.
Normative data report of cycling athletes with percentile scores of Nordic hamstring curl values on NordBord.
Objective Testing During Rehabilitation
When injured, ensuring an optimal return to previous baseline measures and strengths plays a vital role in an athlete’s recovery. Taking a criteria-based approach rather than using generic timelines is one way in which the clinician and team can make calculated objective decisions.
Taking a criteria-based approach rather than using generic timelines [allows] the clinician and team [to] make calculated objective decisions.
Measurements of peak force, rate of force development (RFD) and asymmetry between limbs allow the team to alter and change programs to be more individually specific to the athlete’s requirements.
Taking regular and planned measurements throughout the process allows the team to determine when an athlete is “ready” to progress or even return to competition. This insight not only allows the athlete to return to sport safely but also gives the team and cyclist confidence in the approach.
General Return-to-Train Criteria for Cyclists:
1. Strength Recovery: Less than 10% asymmetry is recommended, especially for lower-body injuries. Tools like the IMTP or dynamometers are used to quantify strength.
2. Functional Tests: Cyclists must pass functional assessments that mimic on-bike demands. These tests include:
- Single-leg squats (for lower-body injuries).
- Isometric core stability holds.
- Balance and proprioceptive assessments.
3. Biomechanical Analysis: Ensure efficient alignment during pedaling. For example:
- No excessive knee valgus/varus during the pedal stroke.
- Symmetrical power output between legs (monitored via power meters or force plates).
4. Injury-Specific Criteria:
- Knee Injuries: Full patellar tracking, no swelling and <10% hamstring and quadriceps strength asymmetry.
- Back Pain: Pain-free trunk flexion/extension, ability to sustain the cycling posture (e.g., aero position) for 20-30 minutes.
- Fractures (e.g., Clavicle or Wrist): Imaging confirms bone healing, with no discomfort during weight-bearing through the arms.
General return-to-train criteria for cyclists, outlining key stages from injury onset to full recovery and return to cycling.
Conclusion
Muscle strength testing in cycling is an essential tool for optimizing performance and safeguarding against injuries. By understanding the key muscle groups, employing effective testing methods and using the data to inform targeted training programs, cyclists can achieve greater efficiency, power and endurance.
Furthermore, the integration of strength testing into rehabilitation ensures that athletes return to their peak performance levels safely and confidently.
Whether you are supporting a World Tour professional or a recreational cyclist, regular strength assessments are vital for building a foundation of long-term success and sustainability in the sport. These evaluations not only unlock opportunities to enhance performance but also play a critical role in fostering healthier, more durable and resilient cyclists.
If you would like to learn more about integrating VALD’s human measurement technology into your organization to optimize strength testing, improve performance and reduce injury risk in cyclists, please reach out here.
References
- Vandewalle, H., Pérès, G., Heller, J., & Monod, H. (1987). Force-velocity relationship and maximal anaerobic power in cycle ergometer and vertical jump tests. European Journal of Applied Physiology and Occupational Physiology, 56(6), 650–656. https://doi.org/10.1007/BF00424805
- Stone, M. H., Sands, W. A., Carlock, J., Callan, S., Dickie, D., Daigle, K., Cotton, J., Smith, S. L., & Hartman, M. (2004). The importance of isometric maximum strength and peak rate-of-force development in sprint cycling. The Journal of Strength and Conditioning Research, 18(4), 878–884. https://doi.org/10.1519/14874.1
- Jongerius, N. (2018). Differences in strength & power profiles between road and time trial cyclists. Journal of Science and Cycling, 7(2), 38–39. https://www.jsc-journal.com/index.php/JSC/article/view/419
- Diefenthaeler, F., Coyle, E. F., Bini, R. R., Carpes, F. P., & Vaz, M. A. (2011). Muscle activity and pedal force profile of triathletes during cycling to exhaustion. Sports Biomechanics, 11(1), 10–19. https://doi.org/10.1080/14763141.2011.637125
- da Silva, J. C. L., Tarassova, O., Ekblom, M. M., Andersson, E., Rönquist, G., & Arndt, A. (2016). Quadriceps and hamstring muscle activity during cycling as measured with intramuscular electromyography. European Journal of Applied Physiology, 116, 1807–1817. https://doi.org/10.1007/s00421-016-3428-5
- So, R. C. H., Ng, J. K.-F., & Ng, G. Y. F. (2005). Muscle recruitment pattern in cycling: A review. Physical Therapy in Sport, 6(2), 89–96. https://doi.org/10.1016/j.ptsp.2005.02.004
- Kubo, K., Kanehisa, H., Kawakami, Y., & Fukunaga, T. (2000). Elastic properties of muscle-tendon complex in long-distance runners. European Journal of Applied Physiology, 81, 181–187. https://doi.org/10.1007/s004210050028
- Blazevich, A. J., Gill, N., & Newton, R. U. (2002). Reliability and validity of two isometric squat tests. Journal of Strength and Conditioning Research, 16(2), 298–304. https://doi.org/10.1519/1533-4287(2002)016%3C0298:ravoti%3E2.0.co;2
- Holliday, W., Theo, R., Fisher, J., & Swart, J. (2023). Cycling: Joint kinematics and muscle activity during differing intensities. Sports Biomechanics, 22(5), 660–674. https://doi.org/10.1080/14763141.2019.1640279